Understanding Quantum Computers and Their Impact on Classical Encryption
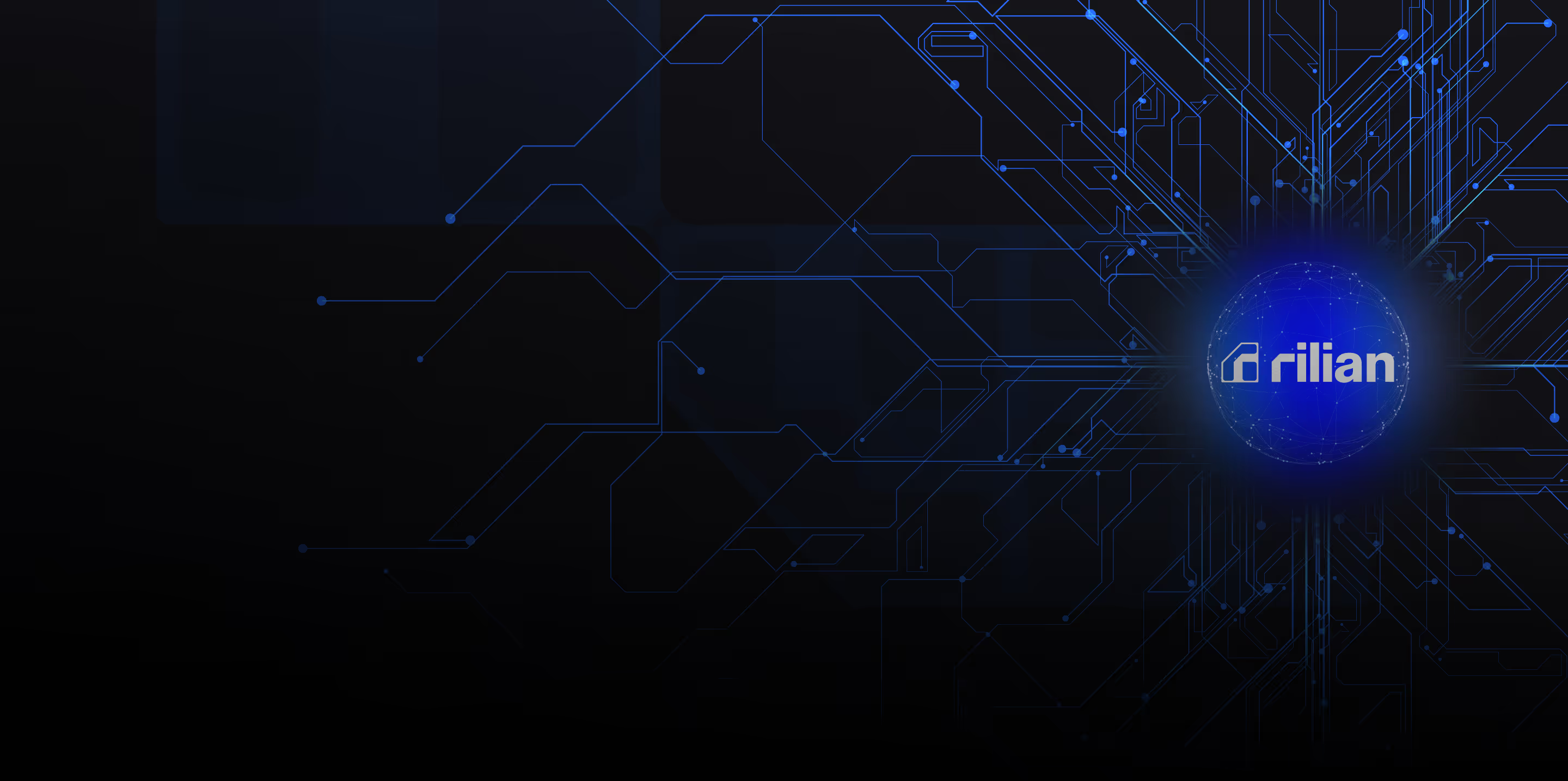
What is a Quantum Computer?
A quantum computer is an advanced type of computer that leverages the principles of quantum mechanics to perform computations. Unlike classical computers that use bits as the smallest unit of data, quantum computers utilize quantum bits or qubits. Qubits have the unique ability to exist in multiple states simultaneously through a phenomenon known as superposition. Furthermore, qubits can be entangled, a condition where the state of one qubit is intrinsically linked to the state of another, regardless of the distance between them. These characteristics enable quantum computers to process complex problems far more efficiently than their classical counterparts.1
Advantages of Quantum Computers
- Advancements in Cryptography and Computational Power: Quantum computers have the potential to outperform classical machines in solving specific types of problems. For instance, they excel at factoring large numbers and solving discrete logarithms, which are the foundation of many classical encryption methods. Through quantum algorithms such as Shor's algorithm, quantum computers can factorize large numbers exponentially faster than classical computers, presenting a significant advancement in fields requiring high computational power.2
- Revolutionizing Quantum System Simulations: Another area where quantum computers demonstrate superiority is in simulating quantum systems. Classical computers struggle to model the behaviour of molecules and materials at a quantum level due to the sheer complexity involved. Quantum computers, however, can simulate these systems natively, opening new avenues in materials science, drug discovery, and chemistry.3
Timeline for Quantum Computing Emergence
The journey toward practical and scalable quantum computing is still ongoing, with researchers and scientists making steady progress. While fully functional quantum computers capable of surpassing classical computers in a broad range of tasks may still be years, if not decades, away, significant milestones have been achieved. Currently, the focus is on increasing the number of qubits and enhancing their stability (known as quantum coherence), which are vital for performing reliable and error-free computations.
Leading companies and research institutions are investing heavily in quantum technology. Google, IBM, Intel, and several startups have made headlines with their advancements, showcasing prototypes with tens of qubits.4 According to experts, achieving quantum supremacy, where quantum computers can perform calculations that classical computers cannot feasibly accomplish, is an imminent goal, potentially within the next few years.5
Current Focus and Challenges
The primary challenges in quantum computing revolve around qubit quantity and quality. Increasing the number of qubits is essential for tackling more complex problems, but each additional qubit introduces more potential for errors. Maintaining quantum coherence, where qubits remain in their superposition state long enough to perform computations, is a significant hurdle. Researchers are developing error-correcting codes and novel qubit designs to mitigate these issues.
Stability and error rates are other critical factors. Quantum systems are highly susceptible to environmental disturbances, such as temperature fluctuations and electromagnetic radiation. Efforts are underway to create more robust and isolated quantum systems to ensure stable operations.
In conclusion, quantum computers hold immense promise for transforming various fields by solving problems that are currently beyond the reach of classical machines. The timeline for their widespread emergence remains uncertain, but ongoing advancements in qubit technology and stability are paving the way for a new era of computation. Transitioning to post-quantum cryptography and developing quantum-resistant algorithms are crucial steps to prepare for the potential quantum future.
Breaking Classical Encryption
Classical encryption methods, such as RSA and ECC (Elliptic Curve Cryptography), rely on the difficulty of certain mathematical problems, like factoring large numbers or computing discrete logarithms. These problems are computationally infeasible for classical computers to solve within a reasonable time frame, thus ensuring the security of encrypted information.
However, quantum computers pose a significant threat to these encryption methods. Shor's algorithm, a quantum algorithm developed by mathematician Peter Shor, can efficiently factor large numbers and solve discrete logarithms, rendering classical encryption techniques vulnerable. A sufficiently powerful quantum computer could decrypt data encrypted with RSA or ECC in a matter of seconds, jeopardizing the security of sensitive information worldwide.6
Examples of where such cryptography is used today include:
1. Internet Communications: Secure websites (HTTPS) use RSA and ECC to encrypt data transmitted between the user's browser and the server. This encryption ensures that sensitive information like passwords, credit card numbers, and personal data remain confidential during online transactions.
2. Email Security: Email encryption services like PGP (Pretty Good Privacy) and S/MIME (Secure/Multipurpose Internet Mail Extensions) rely on classical encryption methods to protect the contents of emails from unauthorized access.
3. Financial Transactions: Banks and financial institutions use RSA and ECC to secure online banking transactions, digital signatures, and secure communications between branches and with customers.
4. Virtual Private Networks (VPNs): VPNs use classical cryptographic algorithms to create secure tunnels for data transmission, ensuring that the information remains private and secure even when transmitted over public networks.
5. Blockchain and Cryptocurrencies: Blockchain technologies, including cryptocurrencies like Bitcoin, use classical cryptographic techniques to secure transactions, create digital signatures, and ensure the integrity of the blockchain ledger.
These applications underscore encryption's critical role in maintaining the security and privacy of our digital communications and transactions.
The "Steal Now, Decrypt Later" Concept
One of the pressing concerns in the context of quantum computing and encryption is the concept of "steal now, decrypt later." This strategy involves adversaries intercepting and storing encrypted data with the intention of decrypting it in the future when quantum computers become powerful enough to break classical encryption algorithms. Even if the data cannot be decoded immediately, it may still be valuable if decrypted later.7
The timeline is critical because encrypted data, especially sensitive information such as state secrets, financial records, and personal data, often retains value over many years. For instance, communications intercepted today could potentially be decrypted in a decade or two once sufficiently advanced quantum computers are available. This makes immediate action imperative to protect current data against future threats.
According to current projections, we may see practical quantum computers capable of challenging existing encryption within the next 10 to 20 years. As a result, the adoption of Post-Quantum Cryptography (PQC) has become an urgent necessity and not just a proactive measure. Organizations and governments need to transition to PQC well before quantum computers reach their full potential to ensure that data remains secure now and in the future.
The Emergence of Post-Quantum Cryptography (PQC)
In response to the potential threat posed by quantum computers, researchers have developed Post-Quantum Cryptography (PQC). PQC refers to cryptographic algorithms believed to be secure against attacks by classical and quantum computers. These algorithms are designed to rely on mathematical problems that are hard for quantum computers to solve, ensuring the continued protection of encrypted data.8
Several candidate algorithms for PQC have emerged, and organizations like the National Institute of Standards and Technology (NIST) are actively working to standardize these new cryptographic methods. In 2016, NIST initiated a call for proposals for PQC algorithms to identify the most secure and efficient solutions. After a rigorous evaluation process, NIST has selected a set of finalist algorithms for further analysis and testing. These include lattice-based, code-based, multivariate polynomial, and hash-based schemes.
Currently, NIST is in the third round of the PQC standardization process, where the finalist algorithms are undergoing extensive scrutiny to assess their security, performance, and implementation characteristics. The goal is to finalize and publish the standards by 2024, providing a robust foundation for the transition to post-quantum security.9
The transition to PQC is a critical step in preparing for the advent of quantum computing and ensuring the security of our digital communications and infrastructures in the future.
In conclusion, while quantum computers hold immense potential for advancements in various fields, they also introduce significant challenges to current cryptographic methods. The development and adoption of post-quantum cryptography are essential to safeguard our information against the powerful capabilities of quantum technologies.
Steps to Take Today for Future Security
Given the imminent threat posed by quantum computing, there are several crucial steps that organizations, governments, and individuals should take today to safeguard their data against future quantum attacks:
1. Educate and Raise Awareness
The first step is to increase awareness about the potential risks associated with quantum computing. Stakeholders at all levels, from executives to IT professionals, should be informed about the vulnerabilities of current cryptographic methods and the need for a transition to Post-Quantum Cryptography (PQC). Educational programs, workshops, and seminars can help disseminate this crucial information.
2. Conduct a Cryptographic Inventory
Organizations should conduct a thorough inventory of their cryptographic assets. This involves identifying all systems, applications, and data that rely on classical encryption methods. Understanding the scope of encryption use within the organization is essential for planning the transition to PQC.
3. Begin Transition Planning
Once the inventory is complete, organizations should begin developing a transition plan to PQC. This includes selecting appropriate PQC algorithms, testing their performance, and integrating them into existing systems. Collaborating with cryptographic experts and consulting guidelines from standardizing bodies like NIST is important to ensure a smooth and effective transition.
4. Implement Hybrid Solutions
While full-scale adoption of PQC may take time, organizations can start by implementing hybrid solutions that combine classical and post-quantum cryptographic techniques. This approach provides an added layer of security and allows for a gradual transition without compromising current operations.
5. Monitor and Adapt
The field of cryptography is continuously evolving, and so are the threats posed by quantum computing. Organizations must stay vigilant and keep abreast of the latest developments in PQC and quantum technologies. To maintain robust security, continuous monitoring and adaptation of cryptographic practices will be necessary.
6. Collaborate and Share Knowledge
Collaboration between organizations, governments, and the academic community is vital for the successful adoption of PQC. Sharing knowledge, best practices and research findings can accelerate the development and standardization of secure cryptographic methods. Participation in industry forums and working groups can help foster a collective approach to tackling the quantum threat.
By taking these proactive steps today, we can strengthen our defences against the quantum computing threats of tomorrow, ensuring that our data remains secure and resilient in the face of advancing technologies.
Citations
- Nielsen, M. A., & Chuang, I. L. Quantum Computation and Quantum Information.
- Devitt, S., Fowler, A., & Hollenberg, L. (2005). Investigating the practical implementation of Shor's algorithm. Proceedings of SPIE - The International Society for Optical Engineering, 5650, 10.
- Ma, H., Govoni, M., & Galli, G. (2020). Quantum simulations of materials on near-term quantum computers. npj Computational Materials, 6, 85. Link
- Arute, F., Arya, K., Bharti, K., Dunsworth, A., Feng, X., Foxen, B., ... & Zhang, L. (2019). Quantum supremacy using a programmable superconducting processor. Google AI Blog. Link
- TechTarget. Quantum supremacy. SearchSecurity. Link
- Zengler, T. (2023, November 7). Quantum computers could break today’s encryption, but there’s hope for the future. Wired. Link
- Cryptomathic. (2020, October 14). How to protect yourself against "steal now, decrypt later." Cryptomathic. Link
- TechTarget. (n.d.). Post-quantum cryptography. SearchSecurity. Link
- National Institute of Standards and Technology (NIST). (2024, August 23). NIST releases first 3 finalized post-quantum encryption standards. NIST. Retrieved from Link
Contact us to know more about our solutions.